Nucleosomes, which consist of DNA wrapped around histone octamers, are the fundamental building blocks of chromatin. Chromatin remodelers utilize energy from ATP hydrolysis to reposition nucleosomes, thereby regulating chromatin structure and gene activity. Chromatin remodelers are divided into four major subfamilies: SWI/SNF, ISWI, CHD, and INO80, each having distinct biological functions. Among them, SWI/SNF enzymes facilitate the formation of open chromatin, widely involved in gene regulation. ISWI senses linker DNA length and drives formation of compact chromatin. Different chromatin remodelers share highly conserved ATP-dependent motor domains, which are central to chromatin remodeling activity. Excessive remodeling must be inhibited to prevent inappropriate disruption of chromatin structure. The mechanisms by which chromatin remodelers overcome histone-DNA interactions to slide nucleosomes are yet to be fully resolved.
On April 4, Science magazine published a study, entitled "Structural insights into chromatin remodeling by ISWI during active ATP hydrolysis", by Professor Zhucheng Chen's team at Tsinghua University School of Life Sciences. Presumably because of the conditions of non-hydrolyzable nucleotides, only three chromatin remodeling states (Apo, ADP, and ADP-BeFx) were captured. This new study employed ATP to sustain DNA translocation, allowing the motor to undergo all possible conformational changes during the remodeling cycle. The researchers designed experiments by varying the ATP concentrations to enrich different conformational states, and used cryo-electron microscopy (cryo-EM) to determine transient structures of ISWI bound to nucleosomes. Nine high-resolution structures were resolved, including ATP, ADP, ADP*, ADP?, ADP*?, Apo, Apo*, ADPS, and ADPB. Based on these structures, the authors propose a comprehensive chromatin remodeling model, which provides mechanistic insights into DNA translocation and linker DNA sensing.
The researchers discovered that ISWI induces a full 1-bp DNA bulge at SHL2 inside the nucleosome (Figure 1). Here, the chemical energy from ATP hydrolysis is converted into the energy of DNA deformation. This conformation, termed ADP*, differs from the canonical ADP state, which exhibits a 1/2-bp DNA distortion. In the ADP* state, the motor induces both DNA strands to shift inwards from the entry side, storing the 1 bp bulge at SHL2 and disrupting the local histone-DNA interactions. These observations contradict two popular models of DNA translocation. The identification of the ADP* state resolves a long-standing question in chromatin remodeling, revealing the mechanism of DNA translocation.

Figure 1. Structure of the ADP* state with a 1 bp DNA bulge.
(A) Heatmap of DNA phosphate backbone displacement. Relative to the ATP state, both DNA strands in ADP* shift inward (arrow direction), and a 1-bp DNA bulge is stored at SHL2
(B) Structural comparison of SHL2 DNA in ATP (gray) and ADP* (colored) states.
(C) Cryo-EM density map of the DNA bulge in the ADP* state.
(D) Analysis of local histone-DNA interactions. ATP state (gray); ADP* state (colored). In ADP*, histone-DNA interactions are disrupted.
The study also identified multiple regulatory conformations of ISWI: ADP?, ADP*?, ADPS, and ADPB. The motors in the regulatory conformations preferentially engage the nucleosome at the side with a shorter linker DNA. ADP? and ADP*? feature positive regulatory elements: RYA (arginine-tyrosine anchor) and PosC (Figure 2A–C), which promote the remodeling activity. In the ADPB state, the positive regulation is lost, and a newly formed Brake element induces an abnormally open conformation, and thus inactivates ISWI. The Brake element is essential for the linker DNA sensitivity of ISWI (Figure 2D–E). Together, these findings elucidate the machinery of linker DNA sensing of ISWI.
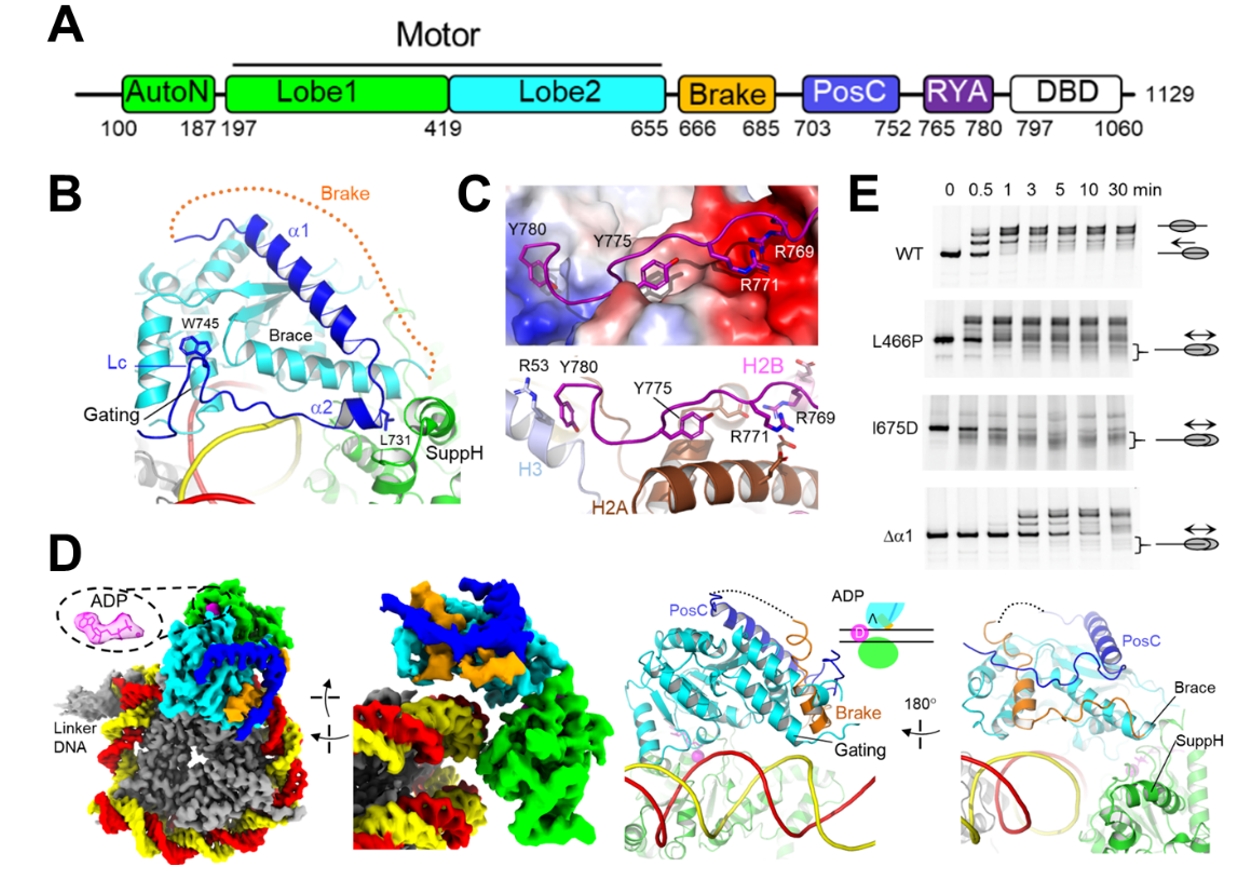
Figure 2. Regulatory conformations of ISWI.
(A) Domain of ISWI.
(B) PosC (dark blue) in ADP?.
(C) RYA in ADP?, with histone surface electrostatic potential shown.
(D) ADPB structure with Brake (orange).
(E) Nucleosome sliding (centering) assay. Wild-type (WT) ISWI senses linker DNA length, sliding nucleosomes to a more centered position (slower gel band). Brake mutants disrupt linker DNA sensing, yielding faster gel bands.
Integrating all nine conformations, the authors proposed a multi-state chromatin remodeling model, which comprises a core translocation cycle and peripheral regulatory states (Figure 3). The core remodeling cycle arbitrarily initiates with ATP hydrolysis and inorganic phosphate release, driving a conformational transition from the ATP state to the ADP state, and induces a 1/2-bp DNA distortion at SHL2. The motor tilts further into ADP*, generating a 1-bp bulge and breaking local DNA-histone contact. ADP releases the Apo* state, and next ATP binding triggers motor closure, propelling the 1-bp DNA bulge propagating toward the exit side.
During the remodeling cycle, DNA deformation in the ADP and ADP* states establish high-energy configurations, representing a critical regulatory node of the remodeling reaction. The motor is stabilized in the ADP? and ADP*? conformations through RYA and PosC, or collapses into the inactive ADPS and ADPB states. The ADPS structure represents a ‘slipping’ state where the energy from ATP hydrolysis does not drive DNA translocation. Short linker DNA diminishes DBD anchorage, inducing the Brake helix formation that locks the motor in an inactive ADPB state. The authors argue that the core remodeling cycle represents a universal mechanism of DNA translocation conserved across chromatin remodelers, while the peripheral regulatory layers are ISWI-specific.
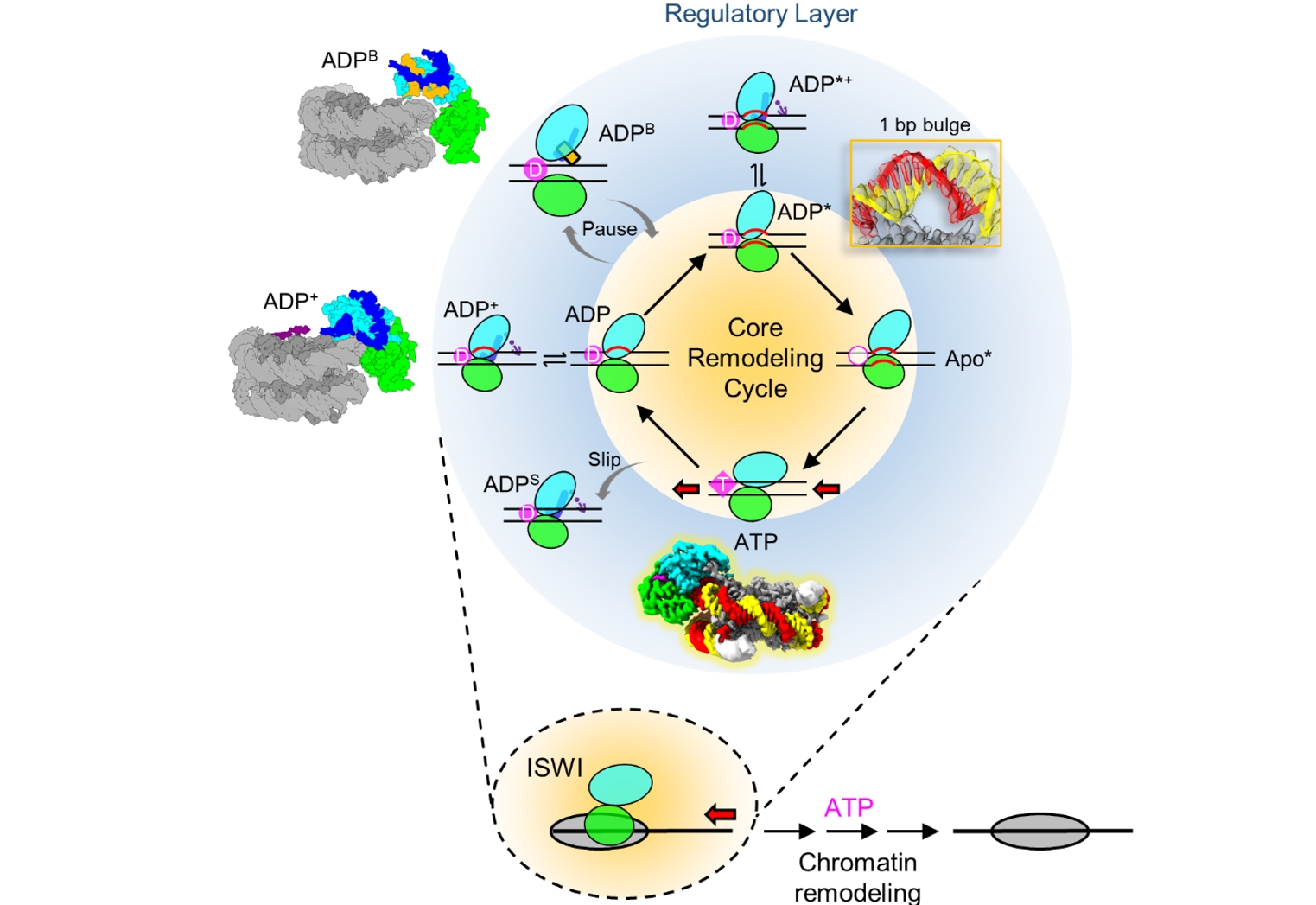
Figure 3. Model of DNA translocation and regulation in chromatin remodeling.
Professor Zhucheng Chen led the study, with PhD candidate Youyang Sia and postdoctoral fellow Han Pan as co-first authors. Postdoctoral fellow Kangjing Chen also contributed to this work. This study was supported by grants from the National Natural Science Foundation of China, the National Key Research and Development Program, and institutional funding through the Tsinghua University Branch of the China National Center for Protein Sciences (Beijing) and Beijing Frontier Research Center for Biological Structure Tsinghua–Peking Joint Center for Life Sciences.
Article link:
https://doi.org/10.1126/science.adu5654
Editor: Li Han